Improvement of Haber-Bosch: Adsorption vs. Absorption
By Trevor Brown on December 01, 2017
At the recent NH3 Energy+ Topical Conference, Grigorii Soloveichik described the future of ammonia synthesis technologies as a two-way choice: Improvement of Haber-Bosch or Electrochemical Synthesis.
Soloveichik, the US Department of Energy’s ARPA-E Program Director, concluded that while new electrochemical technologies promise “potentially lower energy consumption” in the long-term, improvements on the existing Haber-Bosch technology represent the “low hanging fruit” for near-term sustainable ammonia synthesis.
Two of these ARPA-E-funded Haber-Bosch improvement projects also presented papers at the conference. They are taking different approaches to the same problem: how to adapt the high-pressure, high-temperature, constant-state Haber-Bosch process to small-scale, intermittent renewable power inputs. One uses adsorption, the other uses absorption, but both remove ammonia from the synthesis loop, avoiding one of Haber-Bosch’s major limiting factors: separation of the product ammonia.
Ammonia is removed via chemisorption while unreacted hydrogen and nitrogen are recycled. This allows equilibrium limitations to be overcome and can facilitate operation at lower pressure and without the large temperature change exhibited in the Haber-Bosch process.
Palys et al, Design Optimization of a Distributed Ammonia Generation System, 11/02/2017
STARFIRE ENERGY: Fast-Ramping Reactor for CO2-Free NH3 Synthesis
Starfire Energy’s ARPA-E project, Efficient Ammonia Production, was awarded funding through the 2015 OPEN program, in a partnership with Colorado School of Mines.
The Starfire team has already more-or-less completed its fast-ramping reactor, due to be delivered in the first quarter of 2018, and intends to finish building a prototype system, with a production capacity of 3kg ammonia per day, by the second quarter of 2019.
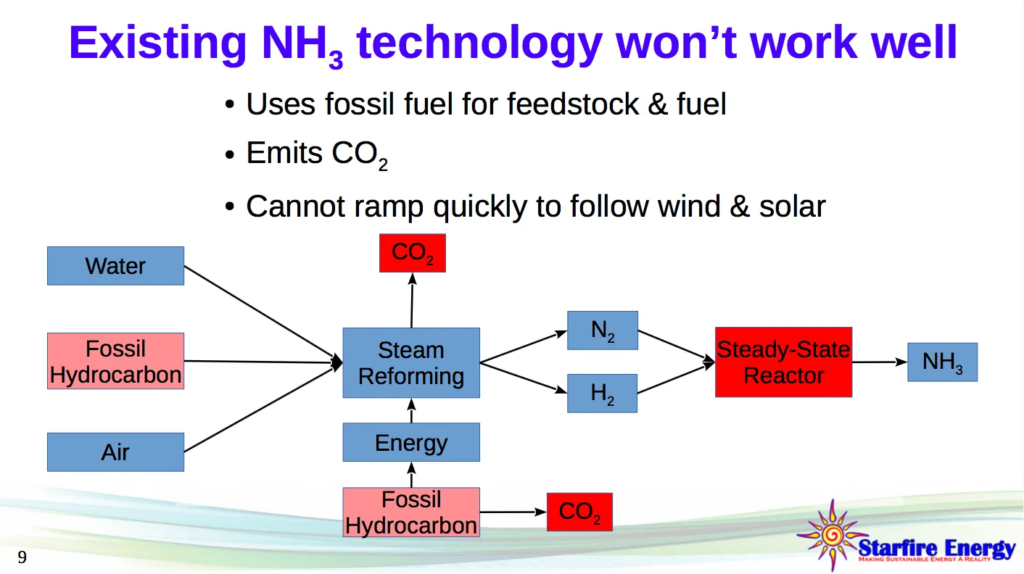
As Starfire’s co-founder, Joe Beach, described it in his presentation at the NH3 Energy+ Topical Conference, the traditional Haber-Bosch process faces two severe disadvantages in the 21st Century energy transition. First, it relies on fossil inputs (natural gas, coal, etc) for both fuel and feedstock, causing significant CO2 emissions (over 1% of global GHGs). Second, it relies on a steady-state reactor, which means that even if the fuel and feedstock were electrified, the ammonia synthesis loop itself cannot “ramp” (turn on and off) quickly: this might not matter for a large-scale, constant power source, like hydroelectricity, but it does not make for an easy match with smaller-scale, intermittent renewable sources, like wind, solar, or tidal power.
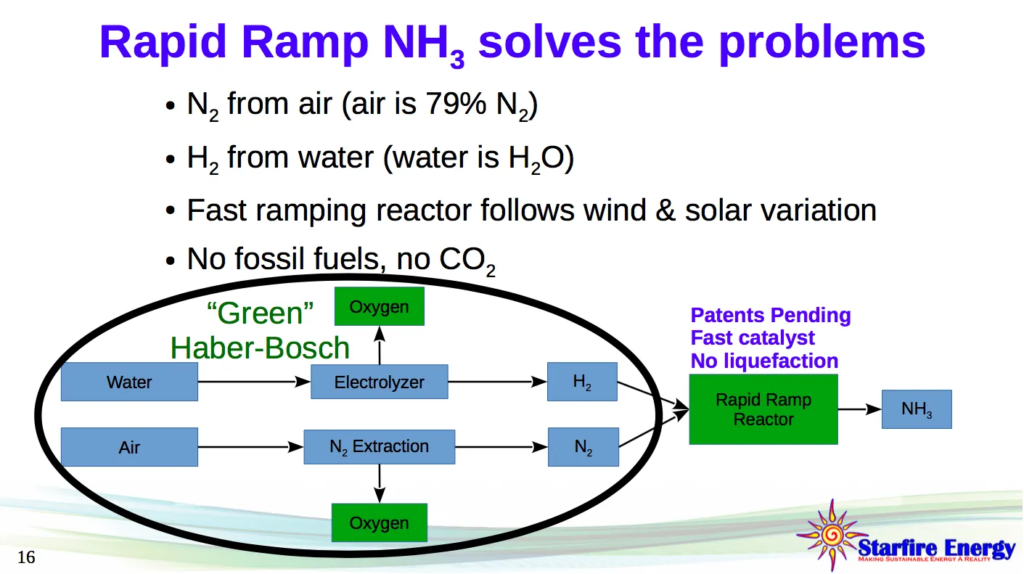
Starfire’s innovation is a fast-ramping reactor that addresses both of these problems. First, it is designed to use renewable electricity instead of fossil fuels (the hydrogen feedstock is produced by water electrolysis) and therefore emits no CO2. Second, it achieves fast-ramping flexibility by combining an improved catalyst with an improved product collection mechanism: adsorption.
Starfire states that its patent-pending, fast-ramping, Ruthenium-based catalyst (“1 wt% Ru on proprietary support”) produces ammonia at ten times the industrial rate, under only one tenth the industrial pressure (“220 mmol/(g·h) at 10 atm,” compared to “Industrial rates: 20 mmol/(g·h) at 100 atm”).
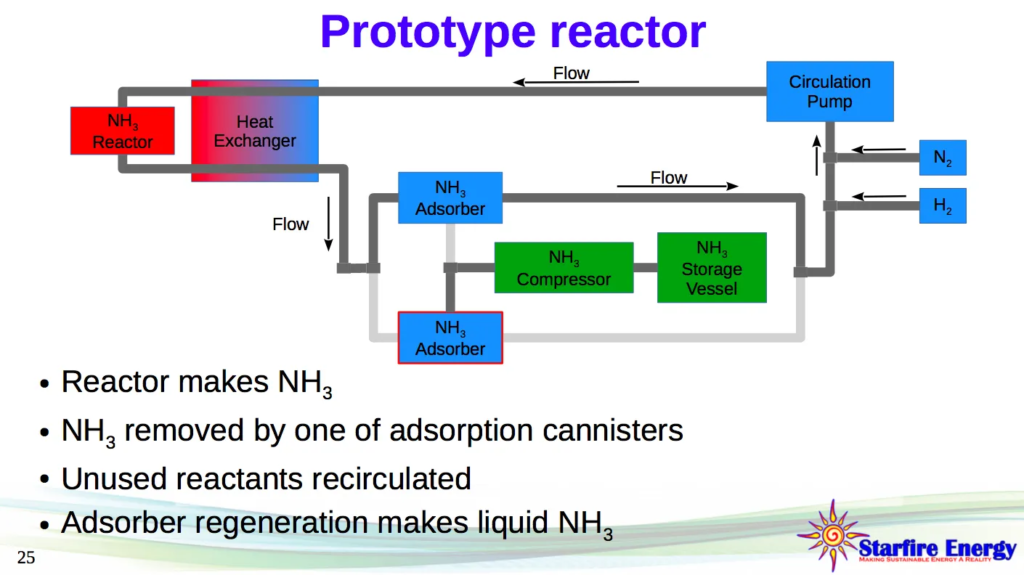
The adsorption system removes ammonia from the synthesis loop, in a reversible chemical reaction. This mechanism overcomes the challenge that “NH3 liquefaction constrains operation” in traditional Haber-Bosch systems, and mitigates the high-pressures and temperature swings.
Starfire’s prototype system includes two adsorption cannisters: at any time, one is removing product ammonia as part of the process flow, while the other is regenerating liquid ammonia for storage.
UNIVERSITY OF MINNESOTA: Lower Pressure Ammonia Synthesis & Design Optimization of a Distributed Ammonia Generation System
Researchers from the University of Minnesota are developing a similar adaptation of the Haber-Bosch system with ARPA-E funding under the REFUEL project, in partnership with NREL, the US Department of Energy’s National Renewable Energy Laboratory. Their ARPA-E project, Small Scale Ammonia Synthesis Using Stranded Wind Energy, builds on years of operational data from the University of Minnesota’s wind-to-ammonia pilot plant in Morris, MN.
This team presented two papers at the NH3 Energy+ Topical Conference. In the first, Lower Pressure Ammonia Synthesis, Mahdi Malmali described the technology improvement and the absorbent they have been developing:
In our reaction-absorption process, we use the conventional catalyst and a solid absorber: hydrogen and nitrogen catalytically convert to ammonia at much lower pressure and absorber removes ammonia from the reactor at high temperature …
Our results indicate that the ammonia production rates can be sustained at pressures as low as 10-20 bar. Our studies show that the ammonia synthesis via reaction-absorption process is no longer controlled by the chemical kinetics or restricted by the reverse reaction, but governed by the absorption of ammonia and the recycle of unreacted hydrogen and nitrogen.
Malmali et al, Lower Pressure Ammonia Synthesis, 11/01/2017
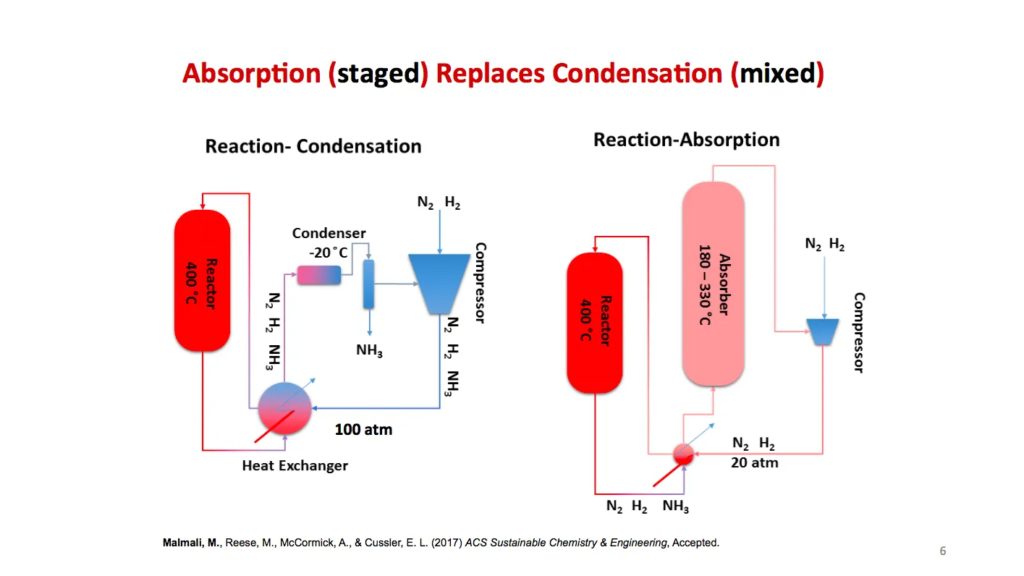
Like Starfire, the University of Minnesota avoids the condensation step, which Haber-Bosch uses to separate product ammonia from the process flow. Unlike Starfire, however, they use absorption not adsorption (for non-chemists this might be an obscure distinction).
The absorbent unit operates at a temperature range from 180 to 330 °C, much closer to the 400 °C temperature of the synthesis reactor. The researchers claim that the absorbent allows for more efficient and complete product separation than in traditional Haber-Bosch plants; it also reduces the need for such high-pressures which, in turn, reduces both operating and capital costs (primarily in heat exchangers and compressors).
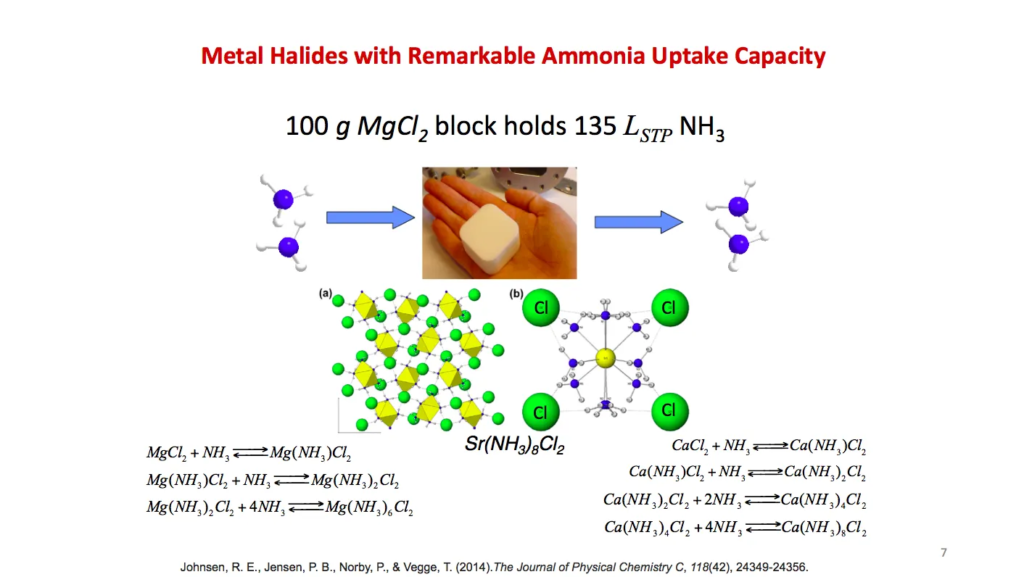
The team provides a full description and evaluation of its reaction-absorption process in a paper, Converting Wind Energy to Ammonia at Lower Pressure, published earlier this month in ACS Sustainable Chemistry & Engineering.
The University of Minnesota team is using metal halides, which have a “remarkable ammonia uptake capacity.” For example, they state that just 100g of metal halide can hold 135 litres of ammonia (“100 g MgCl2 block holds 135 LSTP NH3“).
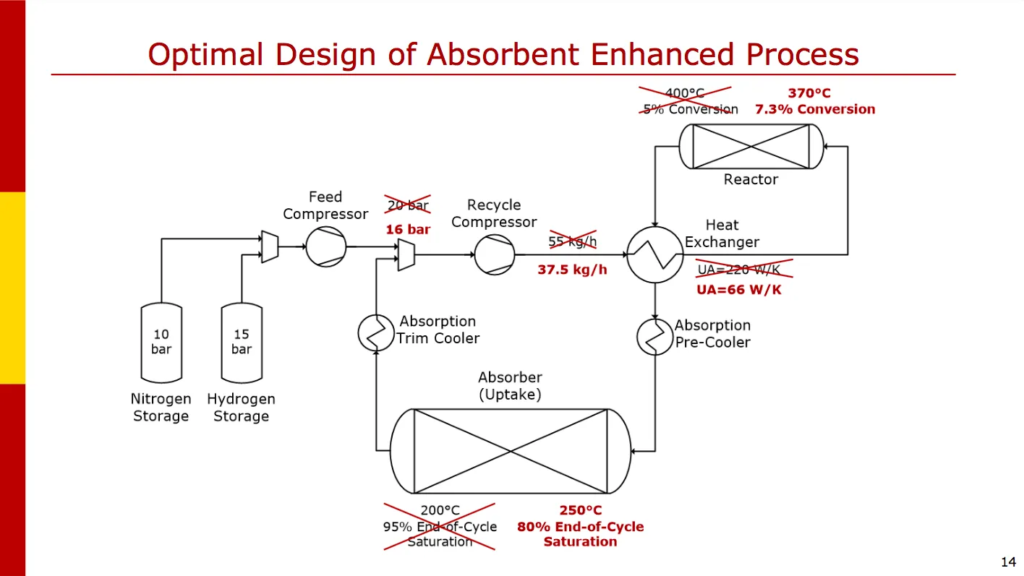
In a second University of Minnesota paper at the NH3 Energy+ Topical Conference, Matthew Palys presented additional work that focused on the optimization of this novel process: Design Optimization of a Distributed Ammonia Generation System.
Technology developments are of greatest value when they can be deployed in the real world, and this second paper demonstrates the extent to which the University of Minnesota’s ammonia program is a unique and globally significant collection of research efforts. It analyses this new process to identify “the economically-optimal design of a distributed ammonia generation superstructure which employs this reactive-separation process. This superstructure uses only renewable energy to provide ammonia fertilizer for a single farm.”
One of Soloveichik’s slides demonstrated a challenge of traditional Haber-Bosch: the vast plant size needed to reach economies of scale, which leads to centralized production far from the consumption centers. The University of Minnesota is optimizing its variant of Haber-Bosch scaled for practical application across the American Mid-West: enabling farms with some of the world’s best wind resources to become self-sufficient producers of their own, reliable, cost-competitive supply of ammonia fertilizer.
Soloveichik’s categorization of ammonia technologies into only two development pathways (Improved Haber-Bosch or Electrochemical) is a useful way to simplify a complicated technology and a varied, globally competitive R&D portfolio. Of course, almost by definition, it doesn’t strictly hold true: even among the ARPA-E REFUEL grantees, there are projects that don’t fit either category. Next, I’ll be reporting on the “misfits” that presented at the NH3 Energy+ Topical Conference: the plasma technologies, process intensification membranes, and solar-thermochemical processes.
You can also read the full article at AmmoniaIndustry.com.