Future Ammonia Technologies: Plasma, Membrane, Redox
By Trevor Brown on December 08, 2017
I wrote recently about two pathways for ammonia production technology development: improvements on Haber-Bosch, or electrochemical synthesis.
Last week, I covered some of these Haber-Bosch improvements; next week, I’ll write about electrochemical processes. This week, I want to write about some innovations that don’t fit this two-way categorization: they don’t use electrochemistry and they don’t build upon the Haber-Bosch process, and that might be the only thing that links them.
These beautiful misfits, which presented their results at the recent NH3 Energy+ Conference, produce sustainable ammonia using non-thermal plasmas, dense metallic membranes, or solar thermochemical redox cycles.
The two-way categorization was put forward by Grigorii Soloveichik, Program Director at the US Department of Energy’s ARPA-E, in his presentation at the NH3 Energy+ conference, and it is a useful simplification – but Soloveichik is well aware that some promising innovations don’t fit into either category. One of the projects he oversees, through the REFUEL program, is West Virginia University’s (WVU) research into Microwave-Plasma Catalytic Synthesis of Ammonia.
WVU’s technology is “a process to convert renewable electricity, water, and air into ammonia using plasma excitation at low temperatures and pressures.” While the WVU team did not present at the recent conference, their ARPA-E project description might help to explain what makes plasma technologies unique and promising:
This process is different from both electrochemical conversion processes and catalytic processes like the HB process. In this form of physical activation, the microwave-plasma process can activate nitrogen and hydrogen, generating ions and free radicals that react over the catalyst surface to form ammonia. Under the correct conditions, microwave heating can selectively heat the catalyst to the temperature required for reactions without heating the surrounding area. This combination of a very hot catalyst and cool surroundings leads to overall lower reaction temperatures and improved energy efficiency. The lower pressure required for the process will also simplify the design. Both features enable better integration with renewable energy sources because the system can be turned on and off more quickly.
US Department of Energy, ARPA-E “REFUEL” project: West Virginia University, Renewable Energy to Fuels Through Microwave-Plasma Catalytic Synthesis of Ammonia, December 2016
UNIVERSITY OF MINNESOTA: Atmospheric-Pressure Synthesis of Ammonia Using Non-Thermal Plasma
The University of Minnesota is also developing a plasma technology for ammonia synthesis, and this team did present at the conference: Atmospheric-Pressure Synthesis of Ammonia Using Non-Thermal Plasma. While the different efforts at the University of Minnesota are clearly coordinated, this is not the team using absorption technology to improve Haber-Bosch, but they are all part of the unique and globally significant collection of research efforts into sustainable ammonia synthesis at the University of Minnesota.
Like the absorption-reaction process, the NTP plasma process has the potential to run at lower temperature and lower pressure, making it possible to operate efficiently at smaller scales, to match renewable power sources like wind or solar farms.
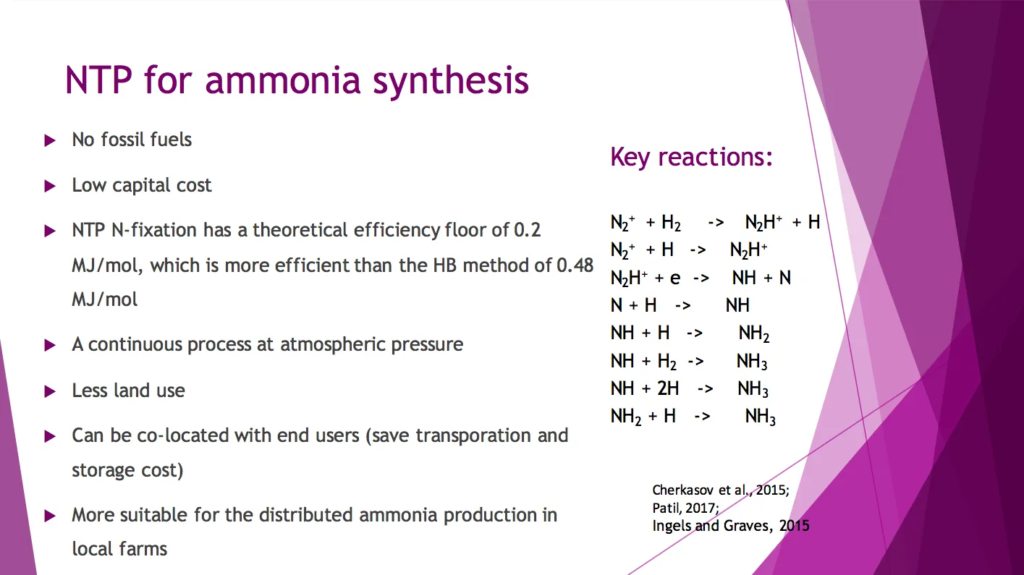
While WVU uses a microwave plasma, which is one kind of non-thermal plasma, the University of Minnesota is using a dielectric barrier discharge (DBD) plasma. They explain the plasma as “Electrically energized matter in a gaseous state, [including] energetic electrons, ions, atoms and molecules, highly reactive radicals, and quanta of electromagnetic radiation (photons).”
It is important to note the technical limits of alternative technologies like this, because it often challenges accepted wisdom. The Haber-Bosch process is mature and, in modern plants, runs almost at its technical limit: no significant further improvements in energy efficiency are possible with Haber-Bosch. This new plasma technology, by contrast, offers significant potential energy savings: “NTP N-fixation has a theoretical efficiency floor of 0.2 MJ/mol, which is more efficient than the HB method of 0.48 MJ/mol.”
The non-thermal plasma (NTP) allows for the synthesis of ammonia at a lower temperature and pressure conditions … the highest ammonia synthesis efficiency achieved was 1.7 g/kWh at 5000V and 26,000 Hz. Results were analyzed in the context of several synergetic effects including the plasma forming mechanism, discharge effects of the catalysts, plasma shielding effects of the mesoporous structure, and other plasma-catalyst interactions. Lastly, a new concept of a two-step dielectric discharge plasma ammonia synthesis method was also proposed, which separates the plasma ionization from the catalytic synthesis and has the potential of improving the NH3 synthesis efficiency.
Peng et al, Atmospheric-Pressure Synthesis of Ammonia Using Non-Thermal Plasma with the Assistance of Ru-Based Multifunctional Catalyst, November 2017
COLORADO SCHOOL OF MINES: Dense Metallic Membrane Reactor Synthesis of Ammonia at Moderate Conditions and Low Cost
Research at Colorado School of Mines (CSM) is also supported by ARPA-E, not through REFUEL but through the program called IDEAS (Innovative Development in Energy-related Applied Science). The CSM project, Low Cost Membrane Reactor Synthesis of Ammonia at Moderate Conditions, makes a simple but significant change to the well-known Haber-Bosch chemical equation:
Instead of:
N2 + 3H2 → 2NH3
Try:
N2 + 6H → 2NH3
In traditional ammonia production processes, nitrogen (N2) and hydrogen (H2) compete for identical catalyst sites, and the presence of each inhibits the other, with the overall rate reflecting a compromise. The team proposes decoupling and independently controlling the N2 and H2 dissociation by dedicating one side of the composite membrane to each. In this way, the catalysts may be individually optimized. Highly effective catalysts have been previously demonstrated for H2 dissociation, and the team’s focus will be on exploring early transition metals which have shown great promise as catalysts for N2 dissociation.
US Department of Energy, ARPA-E “REFUEL” project: Colorado School of Mines, Low Cost Membrane Reactor Synthesis of Ammonia at Moderate Conditions
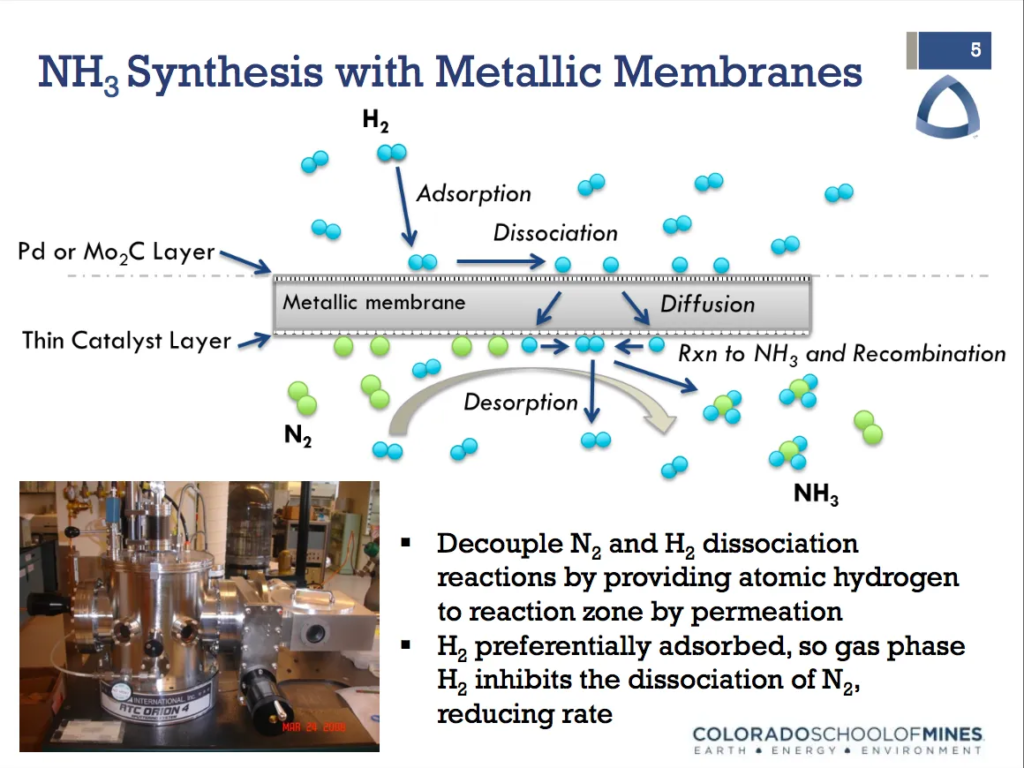
As one of the research leaders explained, “Usually, the membrane is used to remove a product from the reactor, pushing the chemical equilibrium towards products. In our case, we’re using the membrane to provide one of the reactors to the reactor, specifically atomic hydrogen.”
On one side of the membrane, molecular hydrogen (H2) is produced by a reaction like electrolysis or steam methane reformation. On the other side of the membrane, atomic hydrogen (H) feeds the ammonia synthesis reaction. A “process intensification” approach, this technology works by “combining several chemical processing steps into one reactor.”
A new concept for ammonia synthesis has been developed using a hydrogen permeable membrane made from cost effective group V metals. This base metal membrane is coupled with asymmetric catalyst coatings to allow hydrogen permeation and nitrogen dissociation on respective membrane surfaces. The combination of the modified nitrogen dissociation surface catalyst and the highly reactive permeating hydrogen allows ammonia to be formed at low pressures and moderate temperatures. Using this concept, we have measured NH3 synthesis rates as high as 6 × 10-9 mol cm-2 s-1 in preliminary experiments at 500 °C and 0.8 bar.
Fuerst et al, Dense Metallic Membrane Reactor Synthesis of Ammonia at Moderate Conditions and Low Cost, November 2017
KANSAS STATE UNIVERSITY: Nitride-Based Step Catalysis for Ammonia Synthesis at Atmospheric Pressure
At Kansas State, the research team led by Peter Pfromm is working on a step-wise process for ammonia synthesis, using a redox (reduction-oxidation) cycle, and has made significant progress since I first wrote about them at the start of this year.
Unlike a catalytic process like Haber-Bosch, where a catalyst promotes one reaction without being chemically altered, the redox process is a repeating loop of three chemical reactions. A metal, in this case manganese, reacts with nitrogen to form a metal nitride, which then reacts with water to form a metal oxide (and release product ammonia), which then reduces to the metal – and the loop begins again.
Formation of metal nitrides to activate dinitrogen is one avenue to ammonia and other nitrogen compounds. Attractive aspects are operation at atmospheric pressure and moderate temperatures, formation of stable chemical intermediates rather than reliance on somewhat sensitive heterogeneous catalysis, and inexpensive materials.
Heidlage et al, Nitride-Based Step Catalysis for Ammonia Synthesis at Atmospheric Pressure, November 2017
Their goal is to develop a “Versatile, Small Scale Solar NH3 Synthesis” technology, using high-temperature concentrated solar power (CSP), between 600 and 1,000 °C, in a scalable, modular reactor that would enable ammonia production “near the end user … perhaps by a single farmer.”
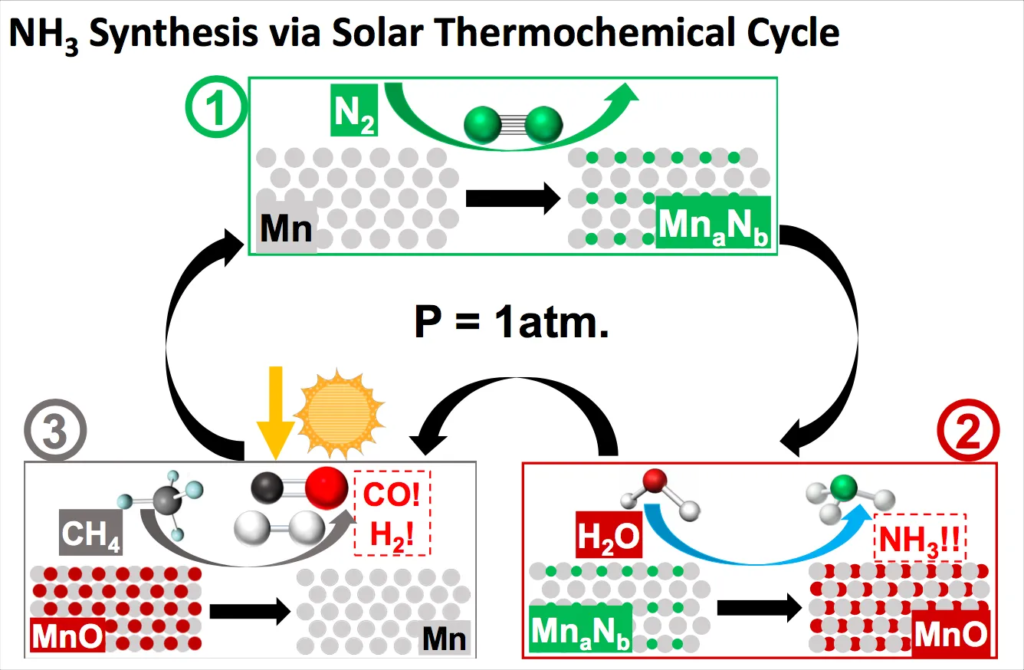
While the CSM membrane technology avoids the nitrogen-hydrogen inhibition at the catalyst by using a two-step reaction (feeding the ammonia reaction with atomic hydrogen), the redox cycle uses a three step cycle. Interestingly, the Pfromm team has also developed a system fed by natural gas (CH4), to co-produce ammonia as well as syngas (carbon monoxide and hydrogen), as well as a system based on a renewable hydrogen input.
UNIVERSITY OF COLORADO, BOULDER: Screening Binary Redox Pairs for Solar Thermochemical Ammonia Synthesis Using Machine Learned Predictions of Gibbs Formation Energies at Finite Temperatures
The Kansas State University technology uses manganese as its metal, cycling between manganese nitride and manganese oxide, but at the University of Colorado, Boulder, researchers are endeavoring to identify the optimal metal to use in a similar redox reaction.
Solar thermochemical ammonia synthesis (STAS) is a reduction/oxidation (redox) cycle which enables the production of ammonia (NH3) from air, water, and concentrated sunlight. In this process, a metal nitride (MN) is oxidized by steam to produce a metal oxide (MO) and NH3; the resulting MO is reduced at high temperature (driven by concentrated solar radiation) and subsequently used to reduce atmospheric nitrogen (N2) and reform the MN and restart the NH3 synthesis cycle.
Bartel et al, Screening Binary Redox Pairs for Solar Thermochemical Ammonia Synthesis Using Machine Learned Predictions of Gibbs Formation Energies at Finite Temperatures, November 2017
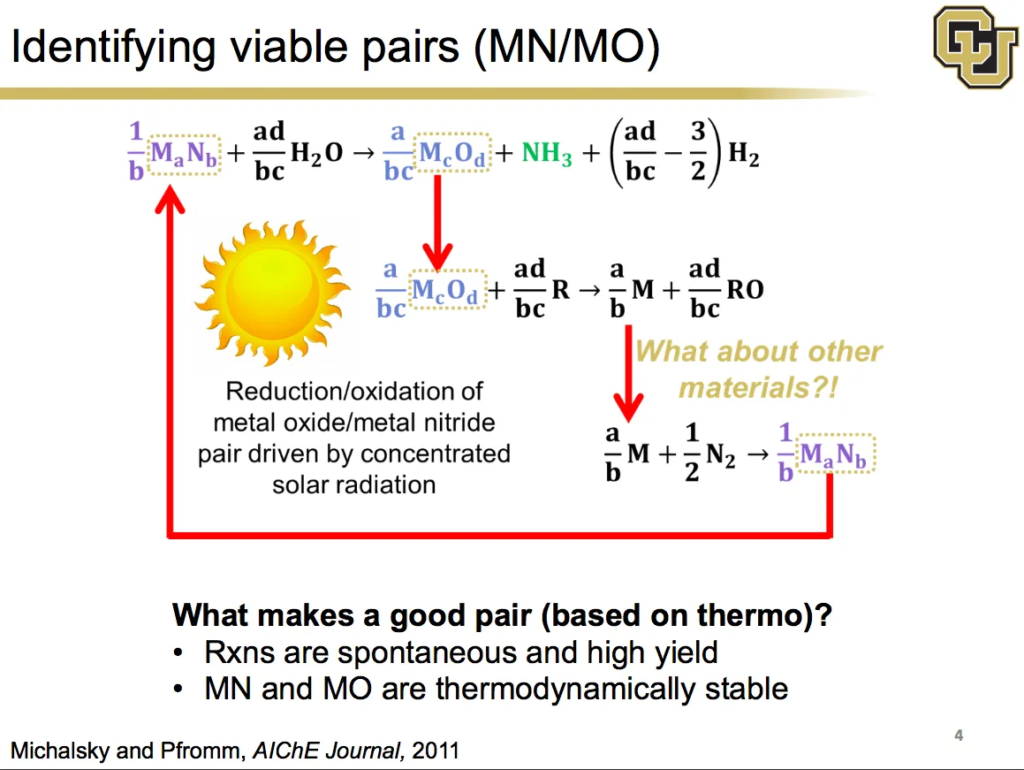
They use machine learning to address the “lack of thermochemical data (i.e., Gibbs formation energies at finite temperatures)” so far established for potential redox pairs, “especially nitrides.” So far, they have “screened the reaction energetics and thermodynamic stability of all known binary (i.e., monometallic) MN/MO pairs, increasing the number of redox pairs considered for this process by an order of magnitude.”
For the skeptics, who believe today’s Haber-Bosch is the best ammonia technology mankind can muster, I would politely remind you of the process to identify the viable catalyst in today’s mature technology, essentially unchanged in 100 years.
Alwin Mittasch, an assistant to Carl Bosch, began the intense, years-long research drive for an optimal catalyst for Fritz Haber’s new ammonia synthesis process in 1909. Over the course of a just few years, he oversaw about 20,000 individual tests and iterations, systematically moving from osmium to uranium and finally to iron, which is essentially what we still use (only decades later did KBR introduce ruthenium).
I imagine Mittasch would have been very glad to have had machine learning tools at his disposal, and I look forward to discovering how far these ammonia synthesis technologies can develop over the next few years.
You can also read the full article at AmmoniaIndustry.com.