Decarbonizing existing, SMR-based ammonia plants: workshop recap
By Kevin Rouwenhorst on November 26, 2023
Future markets for ammonia as a zero-carbon fuel and hydrogen carrier will help spur the development of newbuild, flexible, renewable ammonia plants. Fossil gas-based ammonia at large scale will also be constructed, utilizing autothermal reforming combined with carbon capture and storage (CCS). However, there is already a large fleet of existing gas-based ammonia plants, with an estimated installed global capacity exceeding 150 million tonnes of ammonia. Decarbonizing these existing plants will be as important as building new capacity. During one of the workshop sessions at the AEA’s Annual Conference last November, attendees were invited to help contribute to a “toolkit” for decarbonizing existing SMR-based ammonia plants. Here, we explore some of the material discussed in this session, and some of the proposed pathways for decarbonizing existing, SMR-based ammonia assets.
Upstream methane emissions
Before gas feedstock even enters the ammonia plant, most of its life cycle has already occurred. Steps include:
- Exploration, development and extraction
- Processing and transport of gas
- Transmission, storage and distribution of gas
Methane leakage during extraction, processing and transport can have a significant impact on the carbon footprint of the ammonia product. Methane has a global warming potential (GWP) of about 30 CO2 equivalents on a 100 year horizon, making even small leakages significant.
Upstream emissions can have a significant impact on the overall CO2 footprint of an ammonia plant. Saygin and co-workers estimate that the CO2 equivalent footprint from upstream emissions ranges from 0.19 to 2.40 tons CO2 equivalent per ton of ammonia, with an average value of 0.42. For reference, a state-of-the-art steam methane reformer without CCS emits about 1.8 ton CO2 per ton of ammonia during production, according to the IEA. This means that, if properly accounted for, the impacts of upstream methane leakage could potentially more than double the CO2 equivalent footprint of ammonia.
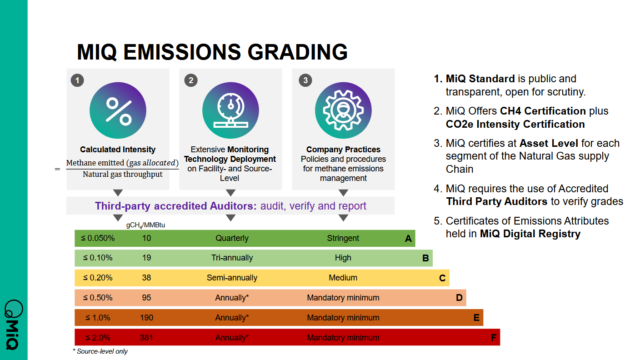
Click to expand. MiQ emissions grading methodology. From Lara Owens, Establishing the Toolkit for Decarbonizing Existing Gas-based Ammonia Plants (Nov 2023).
Sourcing gas with a low-carbon footprint is key for achieving a low-carbon footprint for the produced ammonia. In this context, CF Industries has purchased 2.2 billion cubic feet of gas with 90% lower methane emission intensity versus the industry average, as certified by MiQ.
The MiQ Standard is a public and transparent methodology that allows for determining a grade for the gas feedstock (A is best, F is worst). Grading is based on (1) the calculated methane emission intensity, (2) the monitoring frequency and technology, and (3) company practices for methane emission management. On average, the MiQ-Highwood Index estimates that 2.2% of methane is leaked on average in the United States during the extraction, processing, and transmission of gas feedstock. For reference, MiQ Certified A-Grade gas has a total methane emission of 0.21%.
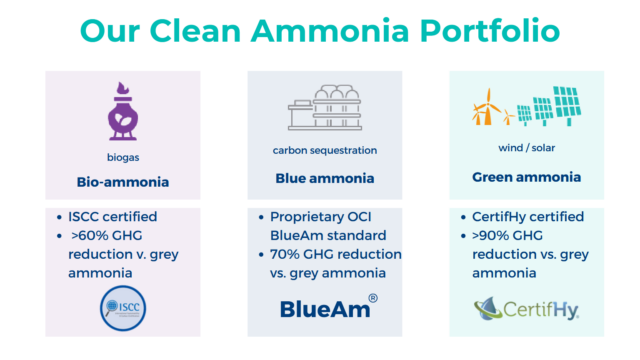
Click to expand. Biogas and carbon sequestration are included in OCI’s clean ammonia production portfolio. Source: OCI Global.
Another strategy is sourcing biogas, which has a reduced carbon footprint owing to its relatively short-cycle CO2. If biogas is combined with CCS, “carbon negative” hydrogen and ammonia can theoretically be produced. It can be mixed with fossil gas to reduce the overall feedstock carbon footprint, and may be a viable pathway to produce urea with a low carbon footprint.
Hydrogen from electrolysis
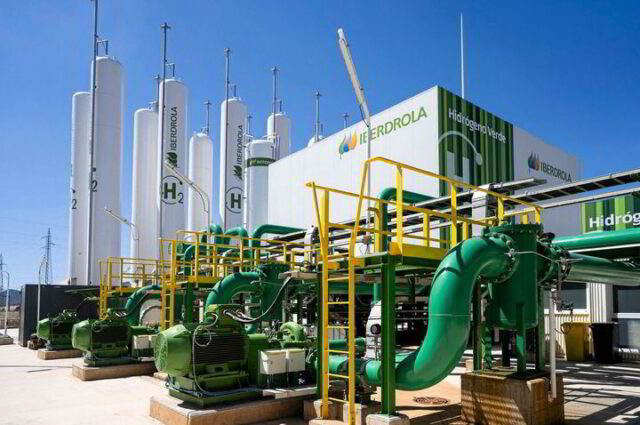
Click to learn more. Electrolysis-based hydrogen plant in Puertollano, feeding ammonia production. Source: Iberdrola.
Another method to reduce the emissions upstream from an existing gas-based ammonia plant is to produce hydrogen feedstock via electrolysis. The electricity powering electrolysis must be from low or zero-carbon sources, such as renewables or nuclear power, to reduce the overall carbon intensity of the plant.
An example of an electrolysis plant added to an existing gas-based ammonia plant is located in Puertollano, Spain. Iberdrola operates a 20 MW PEM electrolyzer facility (Nel ASA technology), which is coupled with a 100 MW solar PV farm and a 20 MWh battery. Hydrogen is stored in 11 pressurized storage tanks. The capacity of this plant is equivalent to about 17,000 tons of ammonia production annually, which amounts to 8.5% of the capacity of the existing gas-based ammonia plant of Fertiberia, the offtaker of the hydrogen.
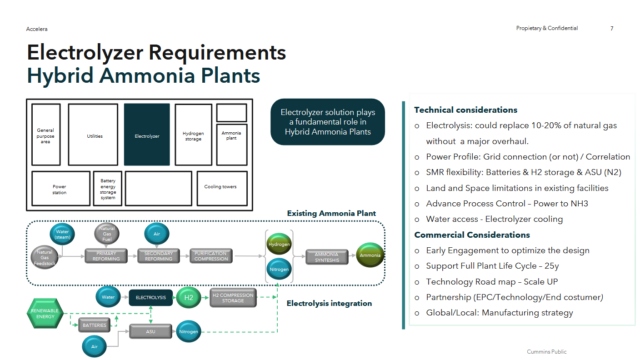
Click to expand. Example of hybrid ammonia plants with electrolyzers. From Francisco Jiminez Garcia, Electrolysis Solutions for Ammonia Production (Nov 2023).
Fully replacing existing SMRs with electrolysis is difficult, given the high degree of heat integration between the ammonia plant and the steam methane reformer unit. Decarbonization beyond 10-20% of an existing gas-based ammonia plant via electrolysis is not possible, unless extensive modifications to heat integration and the steam system are performed. An additional air separation unit would also be required.
Carbon capture & hydrogen burners
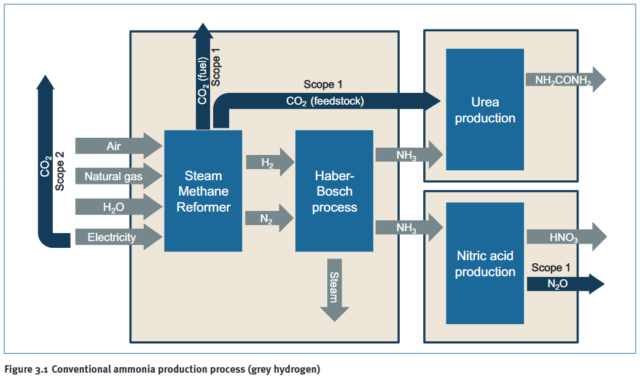
Click to expand. Carbon intensity sources (Scope 1 and Scope 2) for gas-based ammonia production. Source: DECHEMA.
About two-thirds of CO2 in existing gas-based ammonia production is already “captured”. This takes the form of a concentrated CO2 and hydrogen gas mixture produced by the SMR process, also known as “syngas”. All ammonia plants have amine-based carbon capture units (scrubbers) to capture and remove the CO2 from this gas stream, allowing purified hydrogen to enter the ammonia synthesis loop. The captured CO2 can be combusted as a fuel, used as a feedstock or sequestered. One feedstock integration available is urea production. Currently, 55% of globally-produced ammonia is converted into urea.
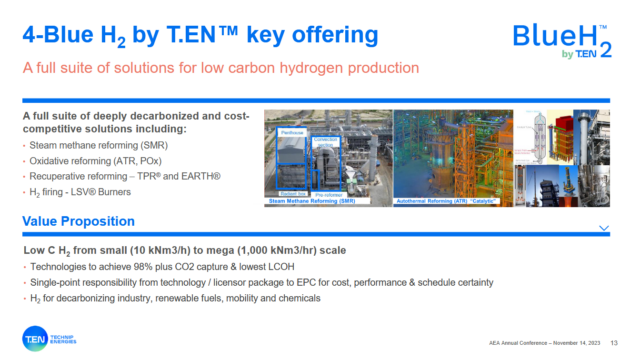
Click to expand. Blue H2 by T.ENTM, a technology for high carbon capture rates from gas-based hydrogen production. From Jeremie Esquier, Toolkit for Decarbonizing Existing Gas-based Ammonia Plants (Nov 2023).
About one-third of CO2 is produced via combustion of fossil gas fuel at the outside of the steam methane reformer tubes, which is currently emitted directly to the atmosphere. Due to the low concentrations of CO2 in the flue gas (typically 18-20 vol.% on a wet basis), carbon capture is more complex and expected to cost about 60 USD per tonne CO2 (according to the AmmonFuel report). Alternative technologies are under development, such as sorbent-based carbon capture that allows for deep decarbonization down to (sub-)atmospheric CO2 concentrations. Thus, a combination of current & under-development technologies may allow to fully remove CO2 from the flue gases. Alternatively, oxyfuel burners can increase the CO2 concentration in the flue gas, making carbon capture easier. Technip Energies has various solutions for carbon capture, claiming an overall carbon capture rate of at least 98% using a mixture of technologies.
An alternative to carbon capture is replacing fossil gas partially or fully with hydrogen fuel. KBR has developed hydrogen-rich burners, as part of its SCOREKlean suite of sustainable designs. KBR has operated 85% hydrogen burners for steam cracking of hydrocarbons for over 15 years. Furthermore, KBR has designed 100% pure hydrogen-fired burners, which have been tested and are being commercialized. KBR’s ammonia cracking technology H2ACTSM will also utilize pure hydrogen burners (instead of hydrogen-ammonia mixtures).
Replacing fossil gas-based burners with hydrogen burners in steam methane reformers decreases the amount of fossil gas required in ammonia production by up to a third. But, this will require additional hydrogen fuel to be produced externally, potentially via electrolysis or electrified steam methane reforming (eSMR). Electrolysis requires electricity and water as feedstocks, whereas eSMR requires fossil gas and steam as feedstocks. eSMR will likely be a relevant option for urea production sites (as concentrated CO2 is a byproduct), while electrolysis is more widely applicable.
Heat storage & electric heaters
Excess high pressure steam from the Haber Bosch process can theoretically be stored as a thermal battery. This allows for the generation of low pressure steam when needed. This strategy is especially relevant for making the ammonia plant more flexible, e.g. by releasing steam for energy when the cost of electricity or gas fuel is high. Yara recently installed a 4 MWh system of ENERGYNEST, which is set to reduce 6,000 tonnes of CO2 annually for its ammonia plant in Porsgrunn, Norway. Such heat storage systems can also be integrated with solid oxide electrolyzer systems, which can be an alternative to water electrolysis technologies like alkaline electrolysis and PEM electrolysis for over-the-fence hydrogen production.
Electrification of heaters and boilers is an alternative to gas-fired heaters and boilers. Interestingly, conventional ammonia plants already feature a “start-up heater”, which only needs to be sporadically used in continuously operating, gas-based plants. However, upon electrification and flexibilization, more frequent operation of a start-up heater may be required to keep heat flows in the process adequate. In anticipation of this, electric start-up heaters have been developed as an alternative to gas-fired start-up heaters.
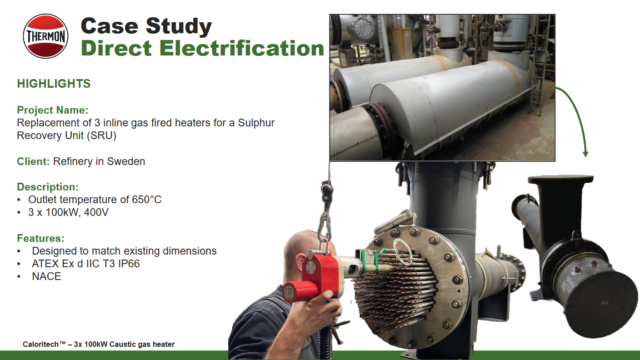
Click to expand. Case study direct electrification by electric heaters. From Dave Yearde, Electric Heating Solutions for SMR-Based Ammonia Plants (Nov 2023).
Electric heaters can also be utilized for on-demand steam production, and for pre-heating gases, such as the units developed by Thermon. This allows for more flexible operation, especially when operating with variable hydrogen supply.
In short, decarbonization of existing steam methane reforming-based ammonia plants is possible, and changes in gas and heat flows can be recovered via alternative technologies. However, the required policy framework and infrastructure for CO2 transportation and storage is not readily available, requiring further attention from policymakers.