Technology options for low-emission ammonia production from gas
By Kevin Rouwenhorst on October 16, 2024
Nearly three quarters of ammonia currently produced worldwide begins with gas feedstock, primarily utilizing the steam methane reforming (SMR) process to produce hydrogen. Low-emission ammonia plants based on gas feedstock are currently under construction in the United Arab Emirates and the USA based on autothermal reforming, an evolution of the SMR process. Other technologies for gas conversion to hydrogen and ammonia are also under development, such as 8RH2, electrified steam methane reforming (eSMR), and methane pyrolysis. In this Technology Insights article, we explore each of these technology pathways, and how they can be deployed for ammonia production.
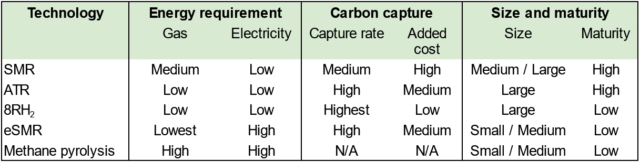
Click to enlarge. Technology options for low-emission ammonia production from gas. * Solid carbon is a product in case of methane pyrolysis, instead of CO2. Solid carbon can be monetized.
Steam Methane Reforming (SMR)
Around 150 million tons of installed ammonia production capacity globally is based on steam methane reforming (SMR). The steam methane reforming process typically refers to the primary reformer in an ammonia plant, wherein gas and steam are converted on a Nickel-based catalyst to hydrogen, carbon monoxide, and carbon dioxide, at 600-900°C and 10-30 bar. The effluent of the primary reformer is further processed in a secondary reformer (a small-scale autothermal reformer, to introduce nitrogen required for ammonia synthesis), and additional hydrogen is produced via the water gas shift reaction (WGS, carbon monoxide and steam are converted to hydrogen and CO2). The CO2 is then removed with an amine solvent.
Click to enlarge. Process scheme for ammonia synthesis based on steam methane reforming (SMR). From Hellmann et al., “Ammonia Synthesis: State of the Bellwether Reaction”, Comprehensive Inorganic Chemistry II (Vol. 7, 2013).
As steam methane reforming is highly endothermic, external energy input is required. Thus, about one third of the gas fed to a steam methane reformer is used as a fuel, resulting in flue gas CO2, which is difficult and expensive to capture. Carbon capture rates beyond 90% for a steam methane reformer are technologically possible, but uneconomical.
CO2 emissions in the flue gas may be avoided in steam methane reformers by utilizing hydrogen burners instead of gas burners. However, newbuild steam methane reformers are unlikely to materialize for large-scale, low-emission ammonia production, given that mature, readily-available alternative technologies have a lower gas consumption.
Autothermal Reforming (ATR)
Autothermal reforming (ATR) technology essentially combines the steam methane reforming reactions with a gas burner inside the reactor, rather than an external burner like in steam methane reforming. The more direct heating in an ATR compared to a steam methane reformer results in a lower overall energy requirement compared to SMR.
Click to enlarge. The Woodside project in Beaumont, Texas (United States), based on ATR with CCS. Source: Woodside Energy.
All CO2 in an ATR is produced in concentrated form, and is captured with an amine solvent. The overall carbon capture rate for ATRs is in the range 93-98%, with an under-construction commercial project in the USA aiming for capture rates beyond 95%.
Numerous low-emission ammonia plants based on ATR with carbon capture and storage (CCS) have been announced, such as in Norway, or are already under construction, such as in the United Arab Emirates, and the USA. Typical single train ammonia capacities are 1-1.3 million tons of ammonia per annum, with an investment in excess of $2 billion.
Technology providers for autothermal reforming include Air Liquide (Lurgi™), Casale & Technip Energies (Blue H2 by T.EN™), Johnson Matthey (LCH™), and Topsoe (SynCOR™). Topsoe offers integrated low-emission ammonia plants based on autothermal reforming, SynCOR Ammonia™. In other cases, technology providers for autothermal reformers and ammonia licensors team up to offer integrated low-emission ammonia plants, such as Air Liquide with KBR, and Johnson Matthey with thyssenKrupp Uhde.
8RH2
8 Rivers has developed the 8RH2 technology. Similar to steam methane reforming, gas and steam are fed to the reformer filled with Nickel-based catalysts. However, rather than gas burners, the 8RH2 technology uses CO2 as the heat transfer fluid in a CO2 convective reformer (CCR). High temperature CO2 is produced in a semi-closed loop similar to the Allam Fetvedt cycle for power generation (wherein syngas and oxygen are co-fed to produce water and CO2 in an oxy-combustor). The CO2 is then fed to the CO2 convective reformer as a heat source for the steam methane reforming reaction.
Downstream of the CO2 convective reformer, air is introduced in the oxygen secondary reformer, after which additional hydrogen is produced via the water gas shift (WGS) reaction, with subsequent purification with a PSA (pressure swing adsorber). The off-gas of the PSA is the feed for the oxy-combustor to produce high temperature CO2.
A benefit of the semi-closed CO2 loop is that CO2 is already available in purified form, without the requirement for amine sorbents to capture CO2. This allows for hydrogen production with carbon capture rates beyond 99%.
Casale is the technology partner of 8 Rivers for scaling up the 8RH2 technology. Project Cormorant, the first commercial-scale project, aims to produce 880,000 tons of ammonia per year in Port Arthur, Texas.
Electrified Steam Methane Reforming (eSMR)
Click to enlarge. Comparison of reactor length scales for gas-fired steam methane reforming and electrified steam methane reforming. From Wismann et al. “Electrified methane reforming: A compact approach to greener industrial hydrogen production”, Science (Vol. 364, 2019).
Decarbonization of heating processes via electric heating is a recent trend for the chemical industry, which can be applied to steam methane reforming. Heating accounts for about one third of the overall energy requirement in a steam methane reformer, implying a significant reduction in gas required in an electrified steam methane reformer (eSMR).
The start-up time for an eSMR is only a few minutes, as compared to hours or even days for the conventional SMRs with gas burners. This allows eSMRs to be added to existing ammonia plants, where they can operate variably depending on the price of incoming electricity.
Click to enlarge. Size comparison for a conventional steam methane reformer plant and an electrified steam methane reformer with the same capacity. Source: Topsoe.
eSMRs can also be used in combination with biogas for low-emission urea production. Using electricity for heating allows for larger volumes of biogas to be converted to the desired product, rather than being used as a fuel. This requires the availability of low-cost electricity on a relatively continuous basis. eSMR technology has been demonstrated for methanol production by Topsoe.
The size of an electrified steam methane reformer is about two orders of magnitude smaller than a gas-fired steam methane reformer. It should be noted that rectifiers and transformers for the electricity supply will still be required, adding to the footprint.
Methane Pyrolysis
Methane pyrolysis is the process for converting methane (CH4) into solid carbon (C) and hydrogen (H2), via electricity input at high temperatures or in a plasma. CO2 is not produced directly in case of methane pyrolysis. Various methane pyrolysis processes exist, with plasma pyrolysis currently the most mature technology.
Click to enlarge. Technology options for methane pyrolysis. Source: sbh4 consulting.
Methane pyrolysis requires about a third more gas compared to SMR or ATR. Also, significant electricity input is required. This comes at the benefit of a valuable solid carbon byproduct: carbon black. The main use for carbon black is in car tires, with the global market for carbon black being around 16.4 million tons in 2022. If all carbon black were produced via methane pyrolysis and all hydrogen from the process were converted to ammonia, this would be equal to around 31 million tons of ammonia production.
Monolith Materials has demonstrated its plasma pyrolysis technology in Nebraska, USA. The Olive Creek I project produces about 5,000 tons of hydrogen per year. Scale-up of the technology in the neighboring Olive Creek II project occurs via module stacking, with 12 plasma pyrolysis reactors in parallel for a total production capacity of 60,000 tons of hydrogen annually. Olive Creek II is currently under construction, and aims to produce 180,000 tons of carbon black each year for car tire manufacturing at Goodyear, plus 275,000 tons of ammonia annually for fertilizer manufacture.